Research Highlight: Jean-Sabin McEwen
Catalysis & Kinetics
Hydrodeoxygenation of Phenolics with Fe Based Bimetallic Catalysts
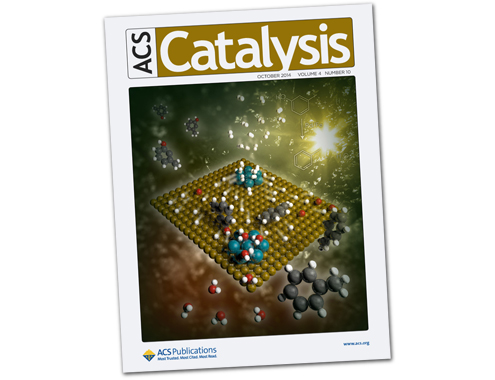
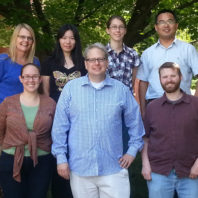
The depletion of fossil fuels, rising fuel costs and corresponding environmental concerns have created an urgent need for exploring green and renewable forms of energy. To this end, the interest towards biomass as a source of fuel has increased rapidly during the last decade. Fast pyrolysis of lignocellulostic feedstocks is considered the most efficient method to produce bio-oil from biomass [1]. The main disadvantage of this method is that the depolymerization compounds (ketones, acids, aldehydes, phenolics) derived from lignocellulosic materials contain quantities of oxygen similar to those of the original biomass (as much as 50 wt% oxygen) [2]. Such high oxygen content causes several undesired properties such as low volatility, corrosiveness, thermal instability and tendency to polymerize under exposure to heat and air. Therefore, there is the need to develop integrative strategies to deoxygenate the pyrolysis products. One particularly promising catalyst for the hydrodeoxydation (HDO) of the derived compounds is a bimetallic PdFe catalyst [3, 4]. The reasons for its exceptional catalytic activity are unclear, however. We propose a unique combination of experiment and theory to address the question of the synergetic interactions within Fe based bimetallic surfaces, since it is only through the combination of our expertise that we can hope to make some headway. Our work to date focuses on two major areas: the adsorption of aromatic molecules on monometallic and bimetallic metal surfaces as well as the enhanced reduction of Fe2O3 with noble metal promoters.
For the noble metal facilitated reduction studies in collaboration with the Wang Research Group, we have studied the adsorption on Pd on an Fe2O3 surface and the subsequent reduction of the surface from both theory and experiment [8]. From experimental temperature programmed desorption (Figure 2 top), the reduction of Fe2O3 to metallic Fe was shown to be significantly enhanced by the presence of Pd on the oxide surface.
The adsorption strength of Pd on Fe2O3 was found to increase with decreasing Pd-O bond lengths (Figure 2 bottom), showing that the noble metal promoter preferentially binds to the surface oxygen. The removal of surface oxygen on clean and Pd covered Fe2O3 surfaces was studied from theory and the results showed that the Pd reduces the energy required to remove surface oxygen as well as thermodynamically stabilize the reduced oxide surfaces. XPS and electronic theory studies showed that the Pd partially donates electrons to the surface Fe which delocalizes the surface electrons and stabilizes the reduced Fe states. This study connected theoretical and experimental techniques in order to fully elucidate the mechanism by which Pd facilitated the reduction of Fe2O3. Overall, this work showed that the Pd partially donates electrons to the surface Fe which stabilizes the metallic Fe state which likely protects the Fe based bimetallic HDO catalyst from deactivation due to oxidation.
[1] G. W. Hubber, S. Iborra, A. Corma, Chem. Rev. 106 (2006) 4044.
[2] R. Maggi and B. Delmon, Biomass Bioenergy 7 (1994) 245.
[3] J. Sun, A. M. Karim, H. Zhang, L. Kovarik, X. Li, A. J. Hensley, J.-S. McEwen, Y. Wang, J. Catal. 306 (2013) 47.
[4] Y. Hong, H. Zhang, J. Sun, K. M. Ayman, A. J. R. Hensley, M. Gu, M. H. Engelhard, J.-S. McEwen, Y. Wang, ACS Catal. Accepted (2014) DOI: 10.1021/cs500578g.
[5] A. J. R. Hensley, R. Zhang, Y. Wang and J.-S. McEwen, J. Phys. Chem. C 117 (2013) 24317.
[6] A. J. R. Hensley, Y. Wang and J.-S. McEwen, Surf. Sci. Accepted (2014) DOI: 10.1016/j.susc.2014.08.003.
[7] R. Zhang, A. J. Hensley, J.-S. McEwen, S. Wickert, E. Darlatt, K. Fischer, M. Schoeppke, R. Denecke, R. Streber, M. Lorenz, C. Papp, H.-P. Steinrueck, Phys. Chem. Chem. Phys. 15 (2013) 20662.
[8] A. J. R. Hensley, Y. Hong, R. Zhang, H. Zhang, J. Sun, Y. Wang, J.-S. McEwen, ACS Catal. Accepted (2014) DOI: 10.1021/cs500565e.